MKIDs are based on a superconducting metal whose electric resistance disappears when it is cooled to low temperatures close to absolute zero. To make use of this property, we keep our MKIDs around a temperature of 0.1 degrees above zero—0.1 Kelvin. When a light ray hits the detector, the metal heats up and its superconducting state changes. This affects the electric current running through, which we observe in the readout signal. MKIDs are ideal to link in a large array because they are easy to read out all at once. To distinguish between pixels, we give each detector a slightly different length so their readout signal has a different frequency.
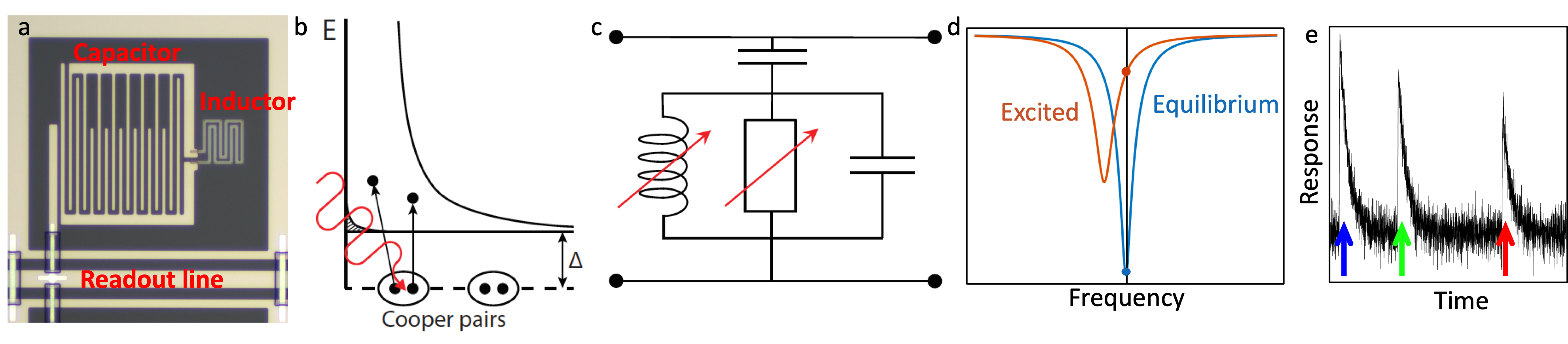
Figure 1: Operation principle of MKID detectors. a) Photograph of one of our MKID pixels. The inductor and capacitor form a superconducting microwave resonator, which is coupled to the readout line. A dielectric lens is placed on top to focus the radiation onto the inductor. b) When a photon is absorbed, it will break up thousands of Cooper pairs into quasiparticles. c) The MKID is a microwave resonator, where the resistance and inductance change upon the absorption of a photon. The change in the resonance curve when a photon is absorbed, which is read out at the equilibrium resonant frequency. d) Response of an MKID to single photons of different colour, which shows the energy-sensitivity of the detector. e) A histogram of measured single photon pulse heights, which demonstrates the state-of-art intrinsic resolving power of MKIDs, here separating different shades of red.