Status | Actief |
Lancering | 2004 |
Ruimtevaartorganisatie | NAOJ |
Type | Ver-infrarood (0,1 – 1 mm) |
Orbit | Atacama-woestijn, Chili |
Instrument met SRON-bijdrage | DESHIMA-2 |
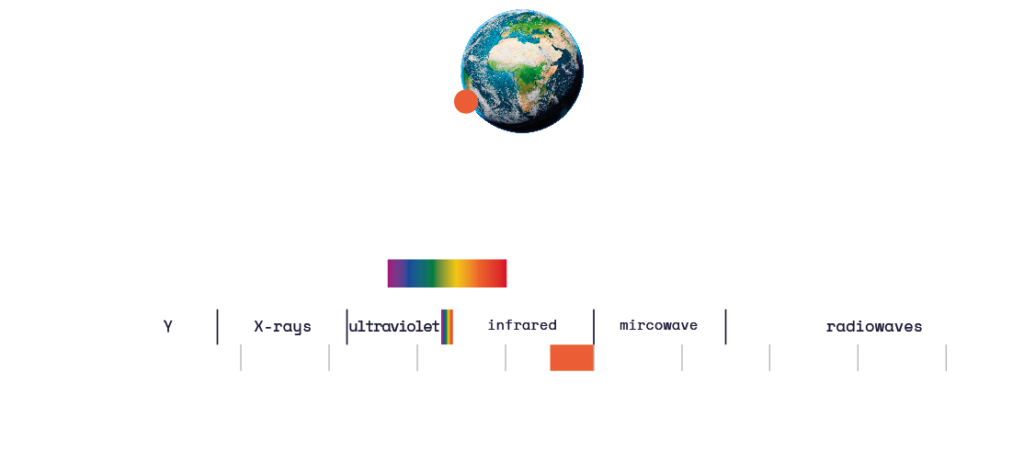
Distances in the universe
Determining distances in the universe is not trivial. The distance to a church tower is still easy to estimate because its size is more or less obvious. But stars and galaxies shine with different luminosities and their size is often impossible to judge. So the brightness of a celestial body says as much about its intrinsic brightness as about its distance from Earth.
Redshift due to the Doppler effect
Astronomers get around this problem by using the Doppler effect. We know this from a police siren that sounds higher and higher until it races past and then sounds lower. Light also has this effect. The redder the light from a galaxy, the faster it moves away from us. Since the universe moves away from every point, this translates almost one-to-one to distance. The light from the furthest and therefore fastest galaxies is so far ‘red-shifted’ that it can be seen on Earth as far-infrared light. Because the light from these galaxies takes the longest to travel to Earth, they show themselves to us as galaxies that have just been born.
Far-infrared
Astronomers interested in these earliest and furthest galaxies, as well as, for example, baby solar systems, are therefore condemned to far-infrared radiation-one of the most difficult wavelengths to observe. Earth’s atmosphere blocks far-infrared radiation with its water vapour while nearby objects act as interferers by emitting far-infrared light as thermal radiation. This is why astronomers seek out the most remote, high and dry places. The Atacama Desert in Chile meets all the conditions.
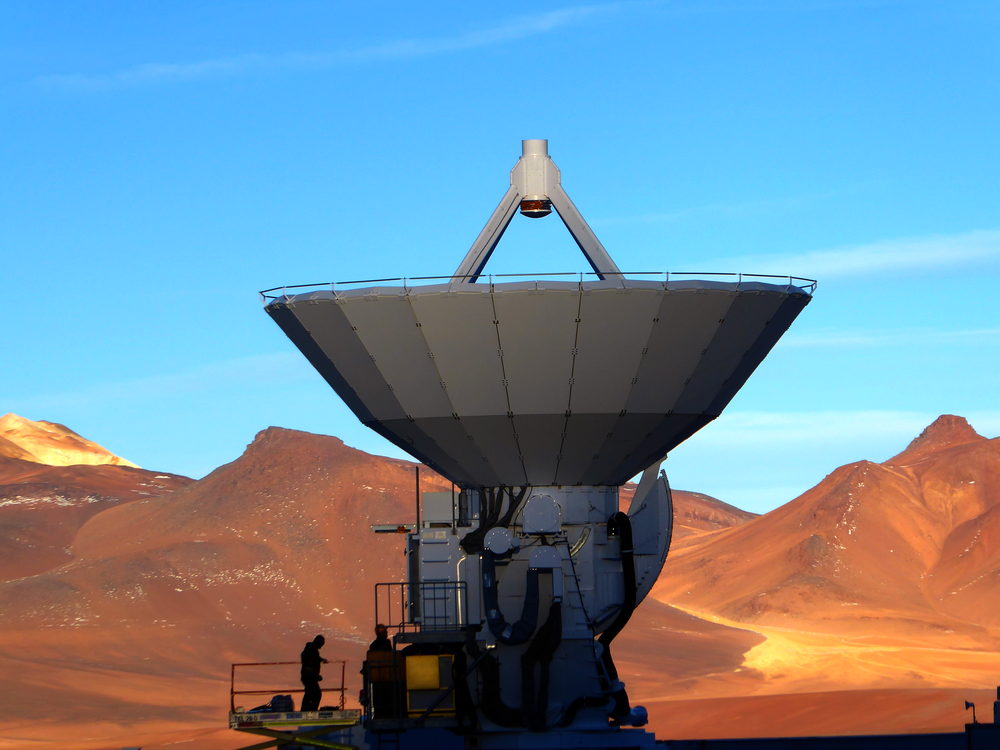
Loss of signal as little as possible
Because the furthest galaxies are so far away and baby solar systems shine so dimly, we have to be sparing with the sparse light that reaches our telescopes, even if we are talking about the 10-metre-wide dish of the ASTE telescope. That is why SRON and TU Delft have developed its detector-DESHIMA-so that it loses as little of the incoming signal as possible. An incident light beam has to bounce back and forth several times to arrive at exactly the right spot in the detector. The researchers tested the successor-DESHIMA-2-on ASTE in 2023. That one loses at most 0.02% signal per bounce.
Kinetic Inductance Detectors
DESHIMA is a Kinetic Inductance Detector (KID), which means it can measure the colour of a light beam by itself, without having to be dispersed by a prism or grating. DESHIMA has a temperature near absolute zero, so it is in a superconducting state. In this state, electrons form pairs, allowing them to flow through the material without resistance. When a light particle strikes, it pierces the material like a bullet, breaking up thousands of those electron pairs in the process. DESHIMA measures how many of these pairs are broken and thus records what the energy was of each incident light particle.
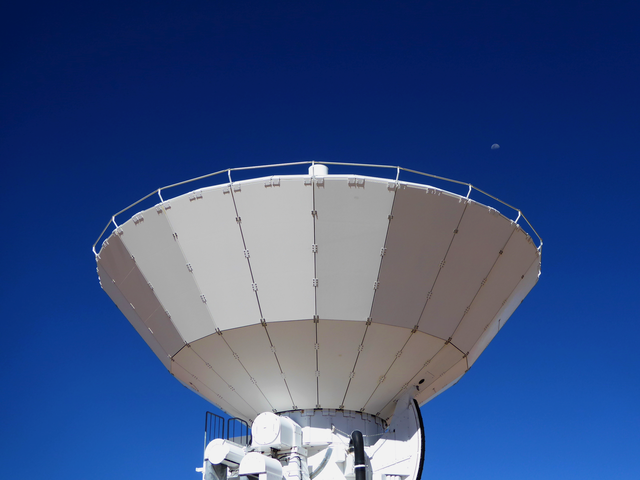